Research
fMRI and the link to neural activity
Recently introduced fMRI techniques provide the ability to visualize elevated neuronal activity with high spatiotemporal specificity and resolution. Such a capability is essential for a system-level understanding of human brain function, which possesses unique attributes and cannot be investigated by the invasive techniques available for animal model studies. However, fMRI signals cannot all be quantitatively related to physiological parameters in a straightforward manner. Understanding the origin and limitations of signal changes detected by fMRI techniques are critical for their full utilization.
Neurovascular and neurometabolic coupling
Since currently available non-invasive methods indirectly investigate brain function by way of vascular or metabolic responses, it is extremely important to understand the relationship of such changes to the underlying neural activity. To investigate such links between neural activity and their associated vascular and metabolic modulations, other techniques, in combination with MRI, are better suited. In our lab, we use modern advances in optical and two-photon microscopy, fluorescent probe development, and optogenetic neuronal stimulation, in addition to traditional methods like electrophysiology and laser doppler flowmetry, to place us at the forefront of studying neuro-vascular and neuro-metabolic coupling and their direct relationship to global and local fMRI signal changes in the in vivo brain.
In vivo imaging of neurodegenerative diseases
Neuroimaging methods are used to investigate neurovascular and neurodegenerative diseases such as stroke, hypertension, Alzheimer’s disease, Parkinson’s disease, glaucoma, etc. Our major interests are to examine early imaging markers, and to investigate in vivo anatomical, metabolic, physiological, and functional changes associated with disease.
Links to Current Projects
- Olfactory Bulb Imaging and fMRI
- Neurovascular Coupling of Cortical Inhibitory Neurons
- Chemical Exchange-Sensitive MRI
- Mouse Imaging of Alzheimer's Disease
Lab Image-of-the-Month Collection
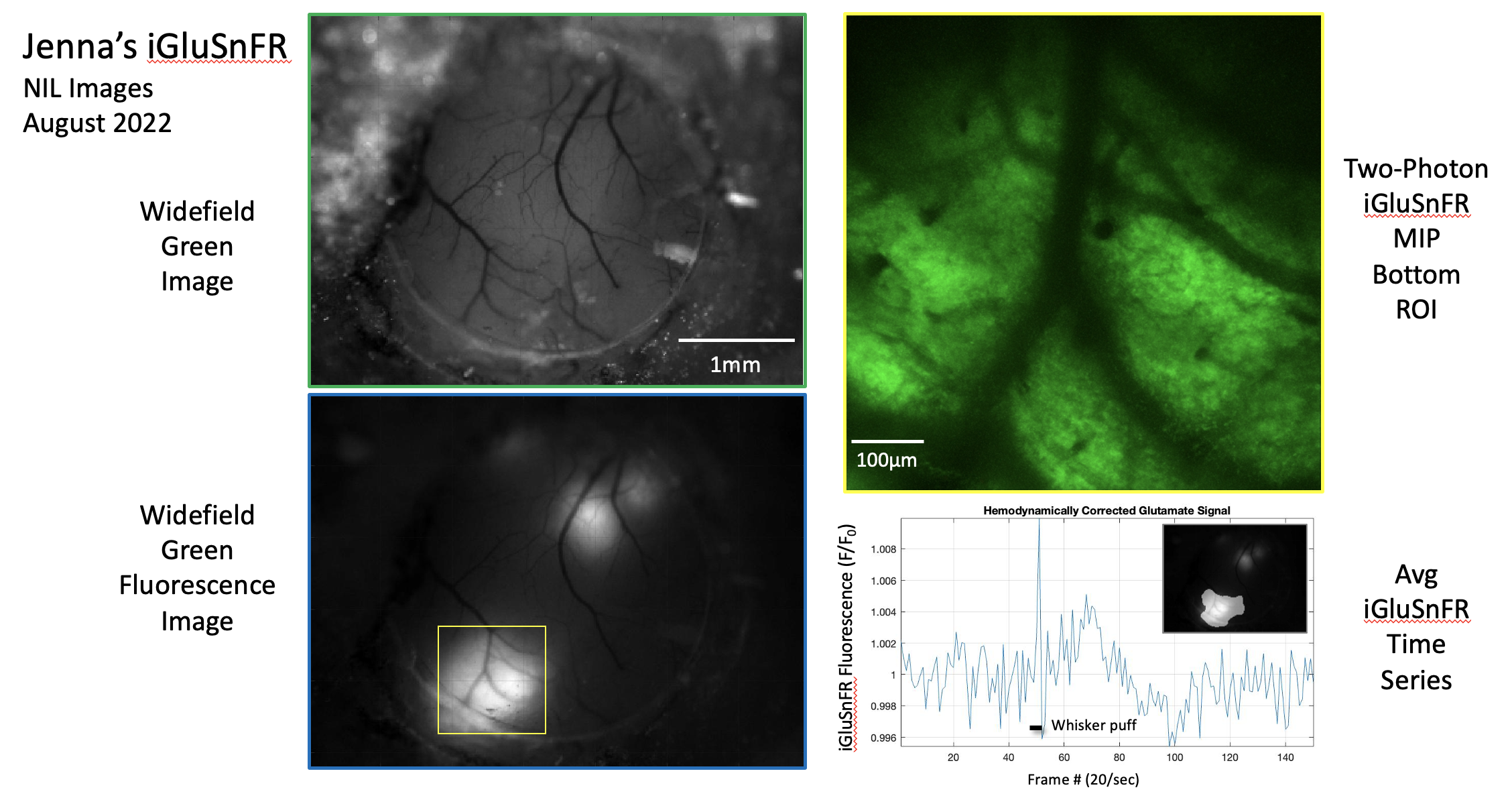

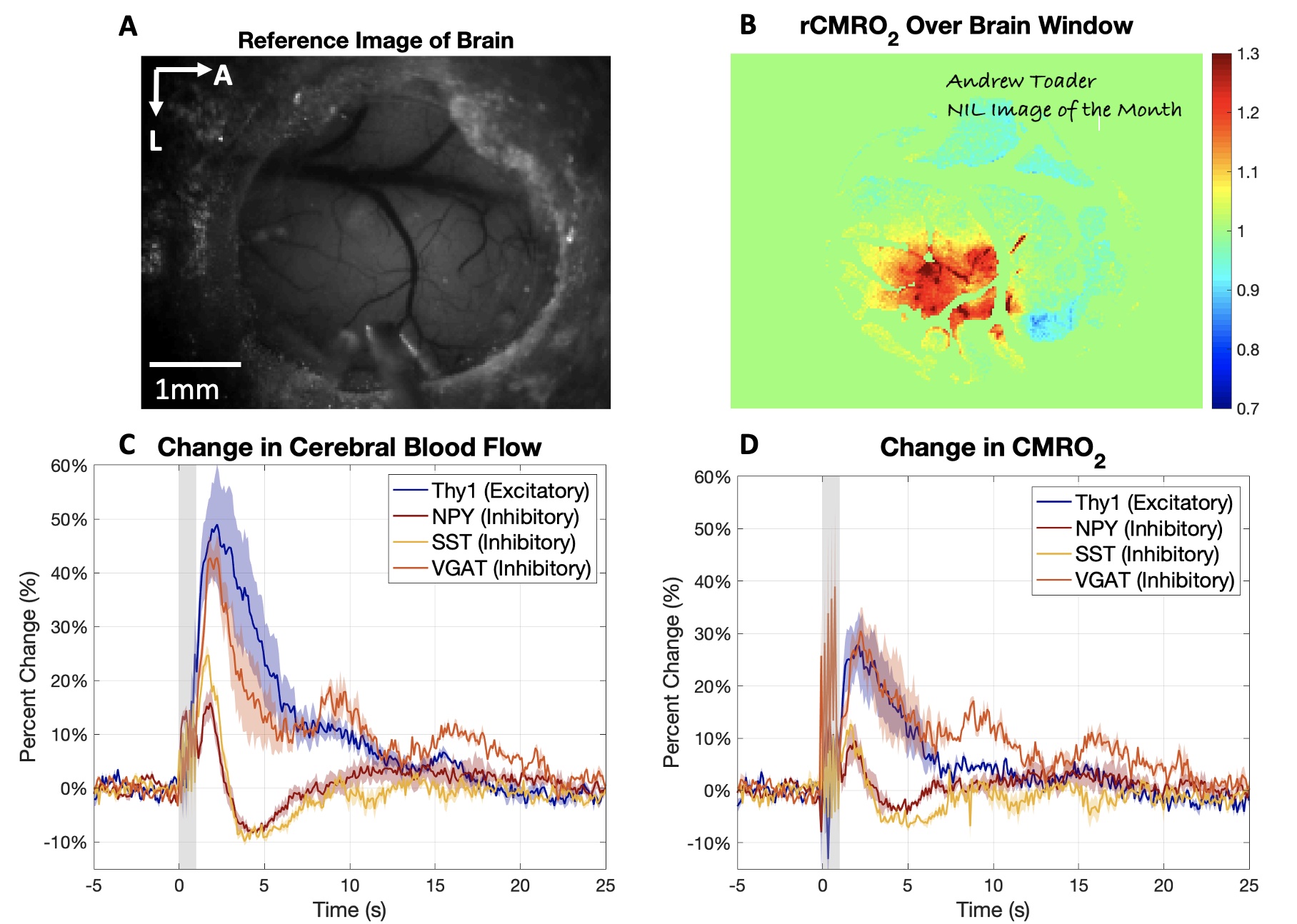

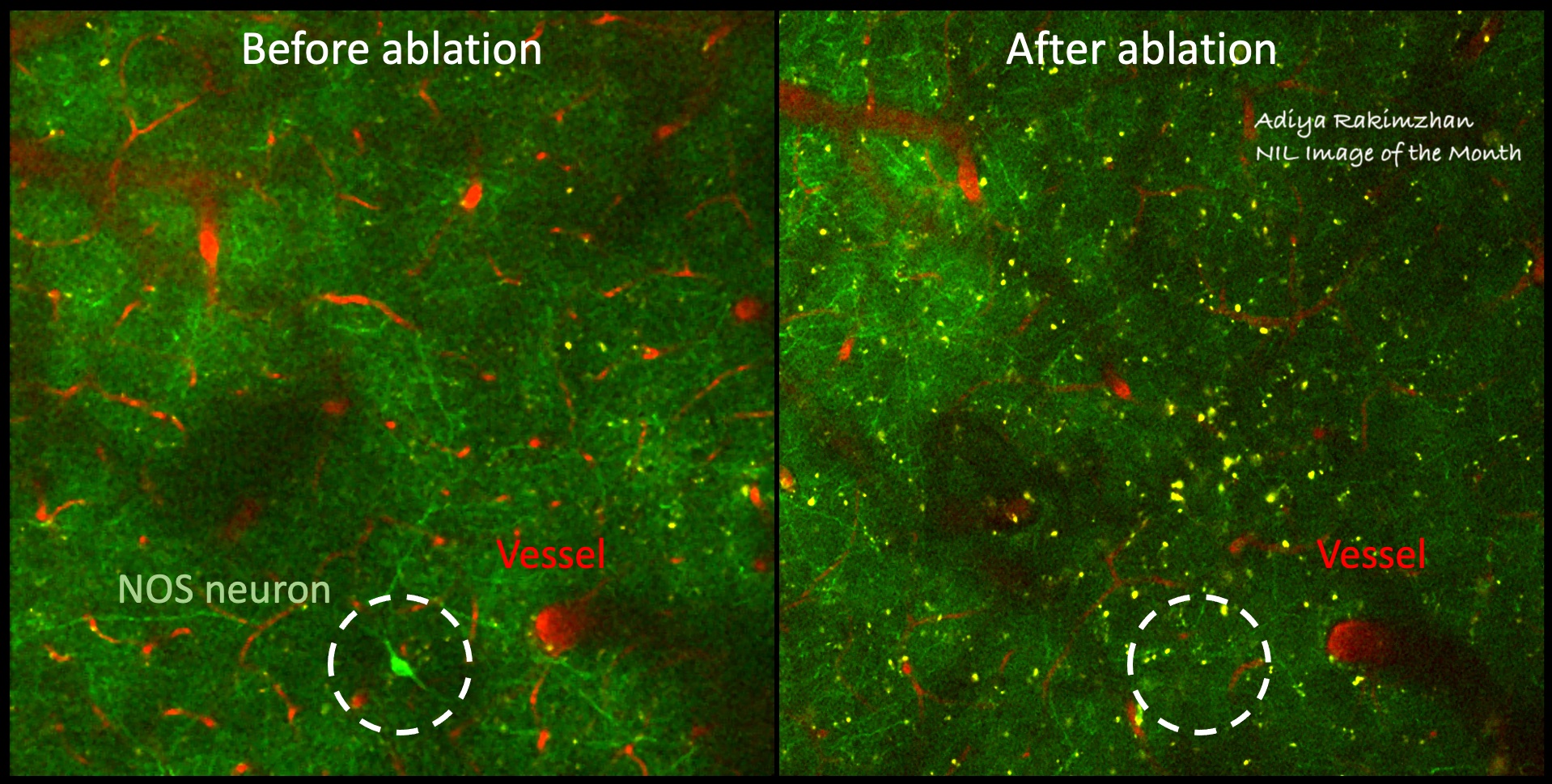
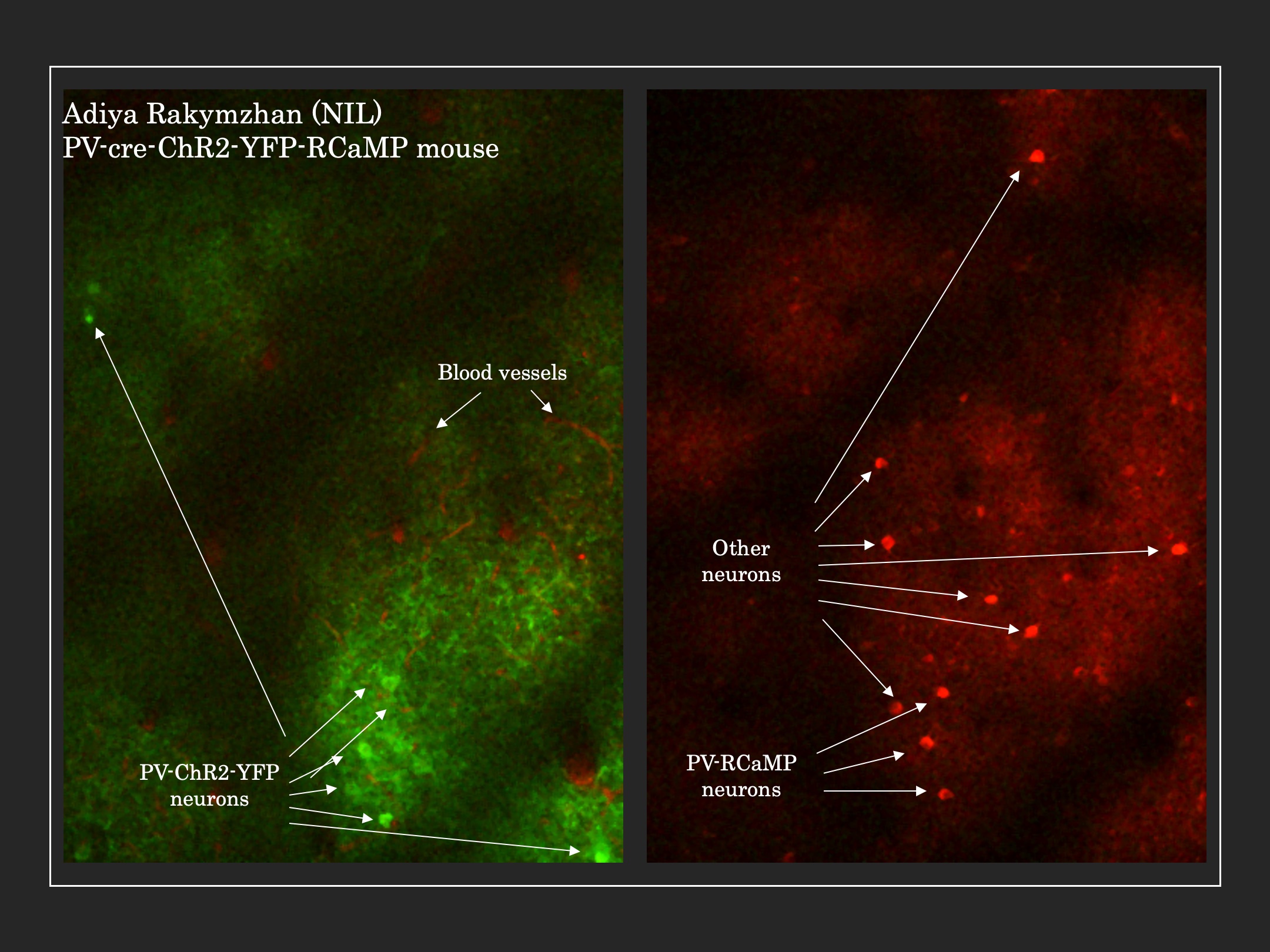
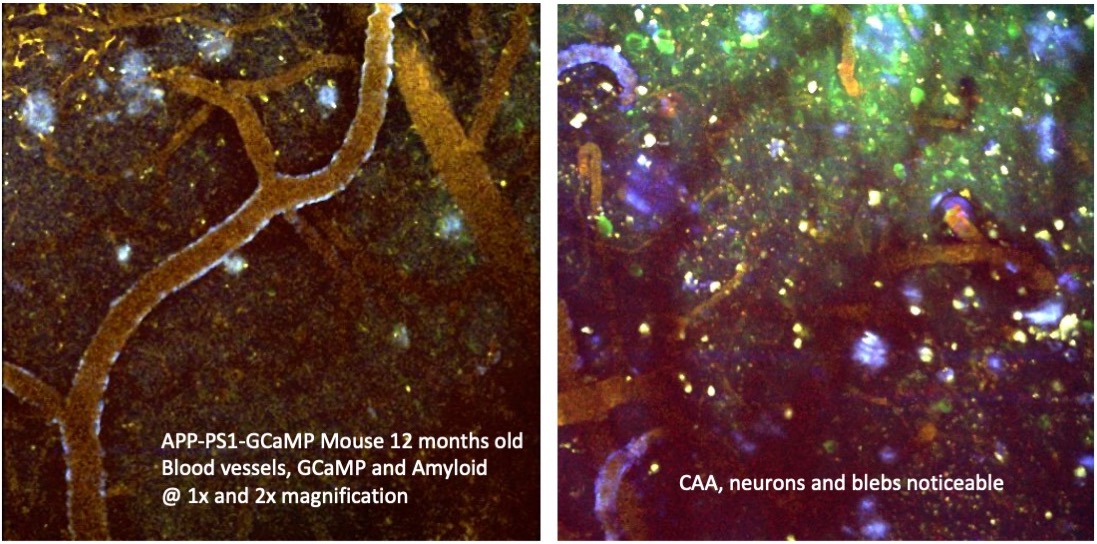